Understanding APD noise and sensitivity
Avalanche photodiodes (APDs) convert light into electrical current. They are widely used in applications where high sensitivity and fast response are required, such as in fibre optic communication systems, laser rangefinders, LiDAR, and optical test equipment.
APDs exhibit internal gain through a process called avalanche multiplication. An applied reverse voltage generates a strong electric field across the diodes’ PN junctions. The generated electrons and holes are accelerated by this electric field. As these carriers move, they gain enough kinetic energy to ionise other atoms in the semiconductor material, creating more electron-hole pairs.
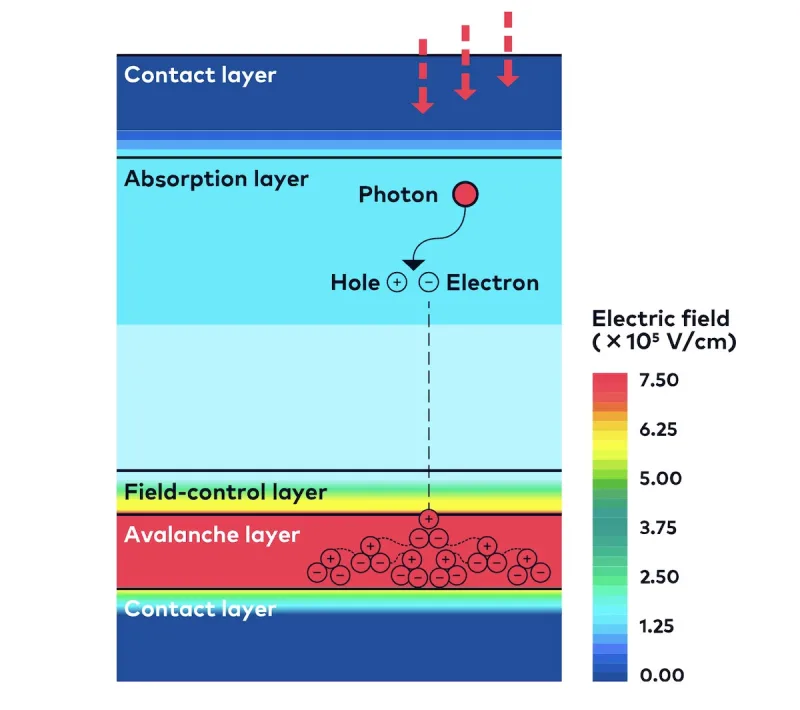
The total generated noise in APDs includes contributions from the following phenomena:
Shot Noise
Shot noise is a result of the discrete nature of charge carriers (electrons and holes) within a semiconductor device. It arises because the dark current or photocurrent consists of a many discrete charges generated randomly within the detector. The discrete nature of charge and its random generation rate time leads to fluctuations in the current. Shot noise increases with the average photocurrent and is influenced by both the primary photocurrent and the multiplied current due to avalanche gain.
Noise from Dark Current
Dark current originates from leakage current within and APD and is present even in the absence of incident light. The contributions to dark current can be divided into “bulk” leakage and “surface” leakage components. Bulk leakage is typically caused by thermal generation of electron-hole pairs within the semiconductor device, however, it can also be caused by carriers tunnelling between the conduction and valence bands. Surface leakage is caused by current flowing across the surface of the device which can be significant if care isn’t taken when designing the device or treating the semiconductor surface. Bulk leakage currents also undergo avalanche multiplication, adding to the noise. Dark current is more pronounced at higher temperatures and limits the sensitivity of the APD, especially in low-light conditions. The dark current generates shot noise, which is typically the dominant source of noise in APDs.
Excess Noise
Multiplication noise is inherent to the avalanche multiplication process within the APD. During avalanche multiplication, the generation of secondary carriers (electron-hole pairs) occurs randomly, leading to fluctuations in the gain. The randomness of the multiplication process causes additional noise factor, known as excess noise. This noise is typically characterised by the excess noise factor, which depends on the multiplication gain (M) and the ionisation coefficients of the electrons and holes. Semiconductors with disparate ionisation coefficients tend to have much lower excess noise factors. The excess noise factor of an APD typically increases significantly with avalanche gain as the electron and hole ionisation coefficients of most materials converge at high electric fields. This limits the useful gain of an APD and degrades the signal-to-noise ratio (SNR).
Thermal Noise (Johnson-Nyquist Noise)
Thermal noise arises from the random motion of charge carriers (electrons and holes) due to thermal energy in the material. It’s present in all resistive elements and is independent of the applied reverse bias voltage. The noise is proportional to the temperature and the resistance of the material. It’s typically constant and does not change with the signal.
Noise limits the avalanche gain in achievable in 1,550 nm InGaAs APDs
For infrared systems operating at 1,550 nm, InGaAs APDs are a popular choice for sensors. However, due to the noise introduced by the mechanisms described above, the multiplication gains achievable without creating an unacceptable SNR, has typically been between M10 and M20.
A new generation of devices known as Noiseless InGaAs® APDs adds an alloy containing antimony to the compound semiconductor manufacturing process. This enables tighter control of the avalanche process and reduces the noise. As a result, Noiseless InGaAs® APDs exhibit 12 times the sensitivity of earlier types. This brings a host of application benefits, for example, in LiDAR, laser range finders, and optical network test equipment. Signals that were previously buried in the noise are now clearly detectable.
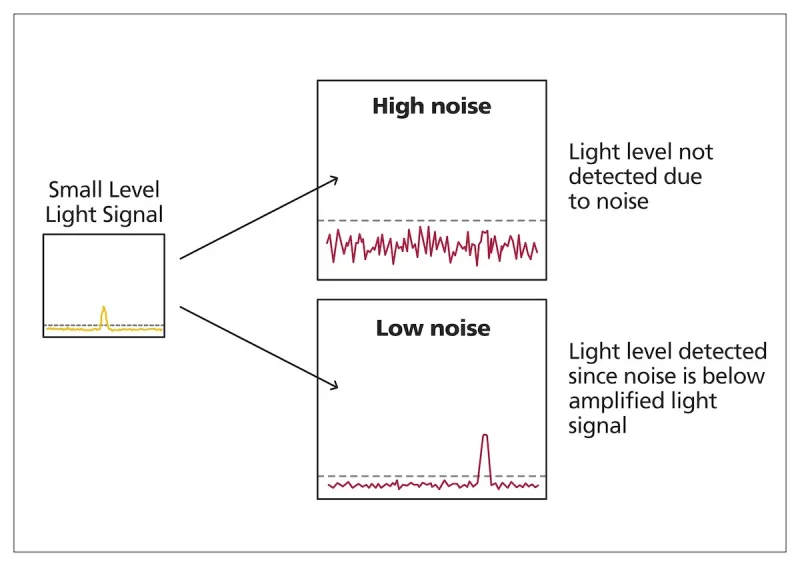
This article looks at Noiseless InGaAs® APD technology in depth and datasheets on the ‘Aura’ family of devices are available here.